Energy, Enzymes and Metabolism
In Lecturio - Metabolism is the sum of both catabolism (pathways that break down molecules for generation of cellular energy) and anabolism (pathways that build macromolecules using cellular energy). Here, we will discuss metabolism with regards to the Laws of Thermodynamics, Enzymology, and go over some of the major metabolic pathways utilized by organisms to perfectly prepare you for upcoming exams.
Table of Contents
Are you more of a visual learner? Check out our online video lectures and start your molecular and cell biology course now for free!
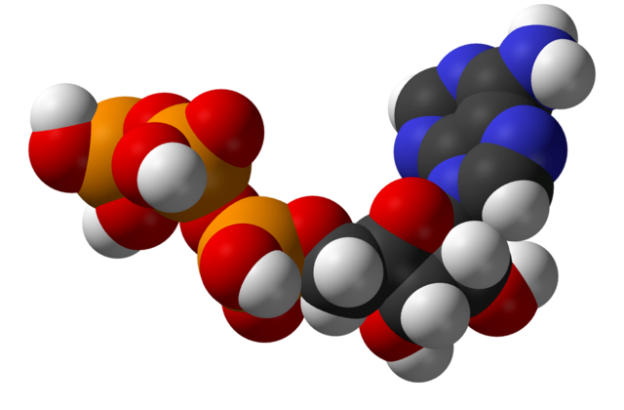
Image : “Structure of adenosine triphosphate (ATP), a central intermediate in energy metabolism” by Benjah-bmm27. License: Public Domain
Cellular Energy
Cells use nucleotide triphosphates, namely adenosine triphosphate (ATP) as their energy currency. As you will see later, coenzymes NADH and FADH2 play a pivotal role in the generation of cellular energy by carrying electrons to the electron transport chain where the concentration gradient of protons is utilized by an enzyme called ATP synthase to generate ATP.Why are nucleotide triphosphates such as ATP a good currency for the cell to use? The answer to that question lies within the structure of the molecule, which consists of a nucleotide molecule, linked by phosphoester bonds up to three phosphate groups. These phosphate groups are stabilized by resonance, and release a lot of energy up their hydrolysis.
You can liken phosphoester bonds on linked phosphate groups to magnets, where if you were to put the like-poled ends together they repel each other. When a phosphoester bond is cleaved, the energy help in those bonds can be used to perform biochemical work, such as transferring a functional group from one molecule to another. This is one way in which enzymes use cellular currency to “pay” for the reactions they catalyze.
Laws of Thermodynamics
The Laws of Thermodynamics describe how enthalpy (heat, q), entropy (disorder, S) and energy (U) behave in inside of a system. Living organisms are open systems, and because of this, from a biochemical standpoint Laws 1 and 2 are the most pertinent.The First Law of Thermodynamics states that energy is conserved. This means that in a biochemical process, energy is not created or destroyed. Energy can be used to perform cellular work, and this change in the energy of a system is described as the difference between the heat (q) that is absorbed by the system from its surroundings and the work (w) done by the system on its surroundings.
ΔU = Ufinal – Uinitial = q – w
The Second Law of Thermodynamics states that entropy (disorder) is always increasing. An analogy that describes this phenomenon is your bedroom, which does not spontaneously clean itself. Energy must be expended in order to go from a dirty (disorder) state to a clean (ordered) state. For a spontaneous reaction, the change in change in entropy is related to enthalpy and temperature as follows:
ΔS ≥ q/T, where T is temperature in Kelvins.
The free energy, G, is what determines the spontaneity of a biochemical reaction. The numerical value of free energy is given by the equation:
ΔG= ΔΗ − Τ ΔS < 0 for spontaneous reactions.
In other words, the value of the free energy change is negative for a spontaneous reaction and is positive for a nonspontaneous reaction. These processes are also termed exergonic and endergonic, respectively.Consider these following four scenarios in determining whether or not a reaction will be spontaneous or not.
- The change in enthalpy is negative and the change in entropy is positive: This reaction is enthalpically favored (exothermic) and entropically favored, and will be spontaneous (exergonic) at all temperatures.
- The change in enthalpy is negative and the change in entropy is negative: This reaction is enthalpically favored, but entropically opposed, and will only be spontaneous at temperatures below T= ΔΗ/ΔS.
- The change in enthalpy is positive and the change in entropy is positive: This reaction is enthalpically opposed (endothermic) but entropically favored, and will only be spontaneous at temperatures above T= ΔΗ/ΔS
- The change in enthalpy is positive and the change in entropy is negative: This reaction is both enthalpically and entropically opposed, and will be nonspontaneous (endergonic) at all temperatures.
Enzymes Catalyze the Reactions of Metabolism
The enzymatic reactions of metabolism must follow the two laws of thermodynamics in sum, whether they are catabolic or anabolic in nature. A given chemical reaction may be endergonic (requiring energy to go from reactants to products) or exergonic (releases energy upon conversion of reactants to products).It is important to note that enzymatic reactions speed up chemical processes that are thermodynamically favorable, by introducing a new pathway from reactants to products. The reaction is sped up because the energy of activation, the hill, is lowered. Enzymes bind and stabilize the substrate in its transition state (ΔG‡) between reactant and product, and this allows for the faster rates observed by enzymatic reactions.
Example: Consider a bag of sugar that has been poured out and left on your kitchen counter. Over a period of thousands of years, the sugar will be converted to carbon dioxide and water. The cells in your body are able to convert sugar to the same sugar to carbon dioxide and water in seconds! How can this be accomplished? The cell does this by using proteins called enzymes, which chemically alter their substrates to form new products.
Biochemical Pathways
The major biochemical pathways of metabolism allow organisms to generate cellular energy by catabolizing macronutrients (proteins, carbohydrates, and lipids) and micronutrients (vitamins and minerals). Cellular energy can then be used to synthesize macromolecules that the organism needs, such as muscle tissue, or stored as energy reserves in the form of glycogen and adipose tissue.A classic example of a biochemical pathway is glycolysisblood as a result of the breakdown of polysaccharides such as liver glycogen, dietary starches, or muscle glycogen. Glucose can also be synthesized from noncarbohydrate precursors in the liver through a process called gluconeogenesis.
In the first step of glycolysis, hexokinase (HK) converts glucose to glucose-6-phosphate (G6P) after it enters the cell through a carrier protein (i.e. GLUT1). Kinases are a class of enzymes that transfer a phosphate group onto their substrates, and in this case the hydrolysis of ATP to ADP+ inorganic phosphate releases enough free energy to drive this otherwise endergonic reaction.
Magnesium is a cofactor needed by the enzyme to shield the negative charges on the phosphate groups of ATP, allowing for hydrolysis of the phosphate and nucleophilic attack on the C6-OH group of glucose. ATP without complexed magnesium is a potent inhibitor of hexokinase. Liver cells contain an isozyme that catalyzes the same reaction, called glucokinase (GK), in order to maintain blood glucose levels.
Phosphoglucose isomerase (PGI) converts G6P into fructose-6-phosphate (F6P) in the 2nd reaction and does not require the input of cellular energy. Phosphofructokinase (PFK) phosphorylates F6P in the 3rd reaction, using a second ATP, to generate fructose-1,6-bisphosphate (FBP). This time, the phosphate on the Mg2+-ATP complex nucleophilically attacks the C1-OH group of the substrate, F6P.
PFK catalyzes the rate-determining step in the glycolytic pathway, and therefore plays a central role in regulating the pathway. The enzyme is sensitive to cellular levels of AMP, which enhance the enzyme, and is inhibited by both ATP and citrate (which “tell” the enzyme that there is an abundance of cellular energy).
Aldolase cleaves FBP into two three-carbon fragments in the pathway’s 4th step, glyceraldehyde-3-phosphate (GAP) and dihydroxyacetone phosphate (DHAP). This reaction does not require cellular energy. GAP proceeds through the glycolytic pathway directly, but the DHAP molecule must first be converted to GAP by enzymatic isomerization catalyzed by triose phosphate isomerase (TIM). This 5th step concludes the energy investment phase of glycolysis, where two ATP were used by hexokinase and phosphofructokinase, respectively. Two 3-carbon molecules were generated from the 6-carbon molecule glucose.
Glyceraldehyde-3-phosphate dehydrogenase (GAPDH) forms the first “high-energy” intermediate in the 6th step of the pathway when its cofactor NAD+ is reduced to NADH. The enzyme places a phosphate group on the carbon in position 1 to yield 1,3-bisphosphoglycerate (1,3-BPG). In the 7th step of glycolysis, phosphoglycerate kinase (PGK) generates the first ATP by converting 1,3-BPG into 3-phosphoglycerate (3PG).
Note that PGK is called a “kinase” because the reverse of this reaction is a phosphoryl group transfer from ATP to 3-phosphoglycerate.
The GAPDH and PGK reactions are coupled, allowing for a slightly unfavorable reaction to proceed in the forward direction. In other words, 1-3BPG conversion to 3PG releases enough free energy to pull the GAPDH reaction forward, even though the GAPDH reaction is endergonic. Another fact to note is that this formation of ATP does not involve oxygen, and is thus an example of substrate-level phosphorylation.In the 8th reaction of glycolysis, 3PG is converted to 2PG by an enzyme called phosphoglycerate mutase (PGM). Mutases are a class of enzymes that transfer functional groups from one position of a molecule to another. Occasionally, 2,3-BPG, which is an intermediate in the mutase reaction, dissociates from the enzyme and binds to deoxyhemoglobin, thereby decreasing its affinity for oxygen. Enolase forms the second “high-energy” intermediate when 2PG is converted to phosphoenolpyruvate (PEP). In this 9th reaction, in which magnesium is used again, water is removed from the substrate. Fluoride inhibits glycolysis by blocking Enolase activity.
The free energy of the hydrolysis of 2PG isn’t sufficient to drive the synthesis of ATP, but the formation of “high-energy” PEP drives the reaction because of its high phosphoryl-group transfer potential in the 10th and final reaction of glycolysis. This reaction is catalyzed by pyruvate kinase (PK), and generates the second ATP by coupling the free energy of PEP cleavage to ATP synthesis in the formation of pyruvate. This enzyme requires both potassium and magnesium to perform its highly exergonic reaction, which releases enough free energy to synthesize ATP.
In the pathway of glycolysis, the 6-carbon glucose molecule is first split into two 3-carbon fragments, using two ATP molecules, and these fragments both generate two ATP molecules in the production of pyruvate.
The net total of ATP production for the pathway is + two ATP. From here, the NADH produced in a pathway can be converted back to NAD+ for further use by the formation of lactic acid, anaerobically; or converted to Acetyl-CoA for use in the Tricarboxylic Acid Cycle (Also called the TCA Cycle or Krebs Cycle) if oxygen is present. The 6th and 10th steps of the pathway (GAPDH and PK respectively) demonstrate how an exergonic reaction can be coupled to an endergonic reaction to “pull” intermediates through the pathway.
Ultimately, the atoms of one 6-carbon glucose molecule will go on to generate 38 total ATP.
Bicarbonate Buffering System
Bicarbonate is an important compound found in human blood. Its capacity to buffer the blood depends on the equilibrium between gaseous carbon dioxide that is dissolved by water and the carbonic acid that is formed by proton dissociation.Maintaining the homeostasis of blood pH is enzymatically accomplished by carbonic anhydrase. As protons are produced by metabolism the bicarbonate-carbonic acid equilibrium will shift toward more carbonic acid. Carbonic acid sheds water and becomes carbon dioxide, which is expired through the lungs. When blood pH rises, more bicarbonate is formed. The rate of breathing is altered so that the increased amounts of carbon dioxide in the lungs are converted to carbonic acid in the bloodstream.
The kidneys are also involved in acid-base balance through the excretion of bicarbonate and ammonium. Patients with disturbances in their ability to buffer their blood properly present with conditions known as…
- …acidemia (blood pH below 7.35).
- …alkalemia (blood pH above 7.45).
Review Questions
The solutions can be found below the references.1. Which of the following statements is true?
- The contribution of ΔS to ∆G increases with increasing temperature.
- The statement that heat always flows spontaneously from an object with a higher temperature to one with a lower temperature can be deduced from the First Law of Thermodynamics.
- All reactions for which ΔS is positive are exergonic.
- All reactions for which ΔH is negative are exergonic.
- The first and second laws of thermodynamics apply only to inanimate, non-living objects.
- Catalysts remain completely unchanged throughout the catalytic cycle.
- Introduction of a catalyst affects both the ΔG<0 and the ΔG‡ for a reaction.
- There is no mathematical relationship between the ratio of forward and reverse rate constants and the equilibrium constant for a reaction.
- Catalysts increase the rate of a reaction in the forward direction but not in the reverse direction.
- Introduction of a catalyst affects the ΔG‡ in both the forward and reverse directions.
- The addition of a catalyst alters the ΔG0 for a reaction without affecting the ΔG‡.
- If one knows the ΔG‡ for a reaction at a given temperature, it is possible to calculate the equilibrium constant for the reaction at that temperature.
- Reactions with positive ΔS0 values are always exergonic, regardless of the value of ΔH0 for the reaction.
- The ΔG0 for a reaction depends only on the identity of the reactants and products, and is independent of the mechanism involved in getting from reactants to products.
- The values of ΔG‡ for exergonic reactions are always negative
Comentários
Enviar um comentário